Farm owners in different places have experienced different problems when it comes to water storage. Over the millennia, agriculture and different empires have flourished from the ability to tap excess water and store it for future use. The ancient empires of Egypt, Israel, Babylon, and even the Chinese empires did this. This is not to say they did not suffer from the challenges that come with earthen embankment construction. With time, and as civilization improved, it became a concern for many how water in such storage facilities would be quickly lost so the secret of Air-bubble Plume destratification seemed to be forgotten. This would result in difficult moments during the dry seasons when crops need water most.
Water loss is not experienced through soil erosion and seepage alone. A study conducted in Australia affirmed the immense effects of evaporation on farm dams. Dams in total contribute to roughly 9% of the total water storage in the country. This amounts to around 7000GL of water and evaporation takes away 40% of this volume on an annual basis. What this does to the small-scale farmers who lack large dams is leave a crippling effect on them. It not only reduces the income to the farmer who relies solely on agriculture but also lowers productivity which leads to food shortages during certain periods in the country. It thus became imperative to find a suitable solution for this.
Applying The DYRESM Air-Bubble Plume Destratification Model
There are many ways to tackle evaporation in dams and lakes. These include placing physical barriers that hinder direct sunlight and even using chemicals that spread on the water facility. Some rely on biological plants that attract animals though this plays a limited role in reducing evaporation in such storage facilities. These methods could still be applied in Australia and still achieve desired results.
Nonetheless, there have been certain constraints in applying these methods in addressing evaporation. Some of these are too expensive for them to be applied on a large scale basis. On the other hand, the inexpensive ones appear to be quite ineffective and reduce only a limited fraction of evaporation rates. In comes the DYRESM air-bubble plume destratification model to combat this evaporative force.
Air-bubble plume destratification is not a new vocabulary in Australia. However, it has been neglected in numerous studies where researchers have placed more efforts in the other methods of reducing evaporation. The DYRESM model appears fruitful and simplistic as it uses already established principles. As such, there is a need for only little calibration and data input. This is because the method has been tried, tested, and validated from different simulations. Moreover, physical limnetic parameters have been included which have stemmed from field and lab studies and proven quite accurate in bringing desired results.
Data that’s mainly needed for the DYRESM Air-bubble plume destratification model to function include;
- Rainfall
- Long and short wave radiation
- Depth-area relationship of the lake understudy
- Wind speed
- Daily discharge of inflows and outflows
- Initial salinity and temperature profiles
- Humidity
- Air-temperature
- Temperature and salinity of inflows.
Most of this data is made ready for you in the daily and sub-daily meteorological readings. It thus becomes easy computing the system based on the Lagrangian Layer Scheme. This presents a reservoir through a scheme of adjoining horizontal layers that have the same properties but vary in number and thickness.
Workings of The DYRESM Model of Destratification
The DYRESM Air-bubble plume destratification model allows for affected layers during outflows and inflows to contract or expand. Similarly, those which are above will either move up or down to accommodate the change in volume.
The principle of this Air-bubble plume destratification model is simple to understand. It works by encouraging the cool bottom water of a lake or dam to come to the surface whereas the surface water goes down. This enables the surface water to cool reducing the likelihood of evaporation. The model is especially suitable in deeper lakes where this can be easily noted. Nonetheless, the study conducted was done in both shallow and deep lakes to differentiate the level of effectiveness in both. It should be noted that the model produced varying results during cooler and hotter periods of the year.
Testing The DYRESM Air-bubble plume Destratification Model
The model had to observe conservation laws for salt, energy, momentum, and temperature that assumes specific heat to be constant and was generalized as:
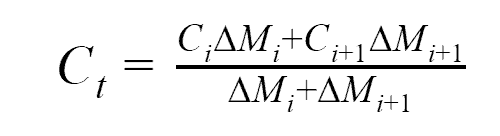
Where:
- C is the property being conserved. This could either be momentum, energy, or salt.
- M refers to the water mass
- The subscripts refer to layer indices.
The main driving factors for the DYRESM model are the momentum exchanges, mass, and surface heat. At the surface, the exchange occurring include the different fluxes and short-wave radiations. The bulk aerodynamic formula is used to describe the sensible and the latent heat fluxes occurring at the surface.
Moreover, DYRESM engages a sub-routine which models the mixing that occurs through destratification devices. These devices include impellers and air diffusers. The working of these devices is based upon established algorithms.
Simulating The DYRESM Model of Destratification
It was obvious that applying the model in a shallow lake would not yield the desired results. That notwithstanding, the researchers wanted to establish just how effective or ineffective the model would be in both a shallow and deep lake. The total period under study was set to 195 days where most of the seasons will be experienced – from September to April.
Logan’s Dam in Queensland is estimated to cover a surface area of 17ha and have a maximum depth of 6.5m. In total, the full water storage capacity of the dam is estimated at around 0.7GL. The South-East Queensland Urban Water Security Research Alliance was so kind as to provide all the meteorological data sets. Another hypothetical dam was used in the simulations having a maximum depth of 13.5m.
The baseline for the simulation and research, in general, is that no destratification or other methods of preventing evaporation was at first used. The DYRESM model would then be later applied for 195 days and the difference observed. The destratification system used composed of a single air-diffuser placed at the bottom of the lakes. It is important to note that the air-flow rates being injected into this system plus the total number of ports on each diffuser have a direct bearing on the results of the system.
The Results after Simulations
In baseline conditions where no method of destratification was imposed, thermal stratification occurred all year round for both lakes. In the shallow lake, this was interspersed with regular periods of weak stratification. For the deeper lake, however, there weren’t any periods of well-mixed water in-between. The surface temperatures varied from a minimum of 18.6℃ to a max of 30.8℃, averaging at 26.2℃.
Baseline evaporation was also quite similar for the two models. An aggregated amount of 897mm for the shallow lake and 901mm for the deep was found to have evaporated. This similarity can be said to be a result of the similarities in boundary conditions.
When destratification is introduced through the air-diffuser, evaporation rates lower in the shallow lake with an increase in air-flow rate. With an increase in the number of ports that varied from 10 to 960, there was also a reduction in evaporation. However, even when all the ports are in use (strong aeration), the evaporation rate only lowers by 1% in this shallow lake. Surface temperature lowers only from 26.2-26℃ in days when bottom temperatures are much colder. However, there is a rise in bottom temperatures from 23.7℃ in baseline conditions to 25.3℃ in the case of strong aeration occurring.
For the deep lake, the reductions reach a level of 5.4% lowering from 901mm to 852mm. At the surface, the temperatures lower from the baseline conditions of 26.2℃ to 25.7℃. However, in hotter periods, the bottom temperatures shoot from the baseline average of 18℃ to 25℃. This significantly increases the heat in the whole reservoir just like in the shallow lake. This means that surface temperatures will eventually increase since destratification is occurring regularly.
Explanation and Conclusion
Differences in surface and air temperatures have a huge role to play in determining the total rate of evaporation. When this difference is high, even after destratification occurs, the cold water brought up will have more to gain from outside heat and because of the sensible heat flux. This means that the destratification process is most convenient during mild weather conditions which can most probably be witnessed during the spring.
As a result, it would not be justifiable to continuously apply the destratification system all-year-round and still expect the same results. This is even worse in shallow lakes where the system is more likely to increase overall water heat thus promoting quicker rates of evaporation at the surface. It is convenient in cool weathers where equilibrium can be attained between the surface and air temperatures. This is only possible if the water beneath is much cooler hence making the destratification technique plausible.
With an increase in heat, the cooler surface water is exposed to sensible heat flux. This generally increases temperatures at the bottom as destratification continually occurs. The only difference between the shallow lake is that the shallow maintains more temperatures even at the surface than the deeper well. As such, the model is convenient in deeper reservoirs especially in cooler periods of the year. Furthermore, DYRESM was an effective tool in not only estimating total evaporation rates in open waters under destratification but also in predicting changes in water temperatures.